13.3 Meiosis
Learning objectives
By the end of this lesson you will:
- Understand how meiosis starts with one diploid cell and results in four haploid cells.
- Know how meiosis produces gametes that are genetically diverse.
- Be able to model the stages of meiosis.
- Be able tompare the similarities and differences in the mechanics of mitosis and meiosis.
Review of sexual and asexual propagation
As seen earlier, there are two broad categories of plant propagation: sexual and asexual. When new plants are produced from existing plant parts, like pieces of leaf, stem, or root, reproduction is asexual and the only type of cell division that has taken place is mitosis, where one diploid cell produces two identical diploid cells.
If new plants are instead produced from seeds, this is a strong indication (but not a certainty…optionally read about apomixis) that reproduction was sexual. Plants that practice sexual reproduction use mitotic cell division when increasing the diploid vegetative parts of the plant like stem, leaf, and root, but use meiotic cell division to initiate the haploid stage of the plant that ultimately results in production of egg and sperm cells central to sexual reproduction. Instead of two diploid cells from one diploid cell (the outcome of mitosis), the outcome of meiosis is four haploid cells from one diploid cell.
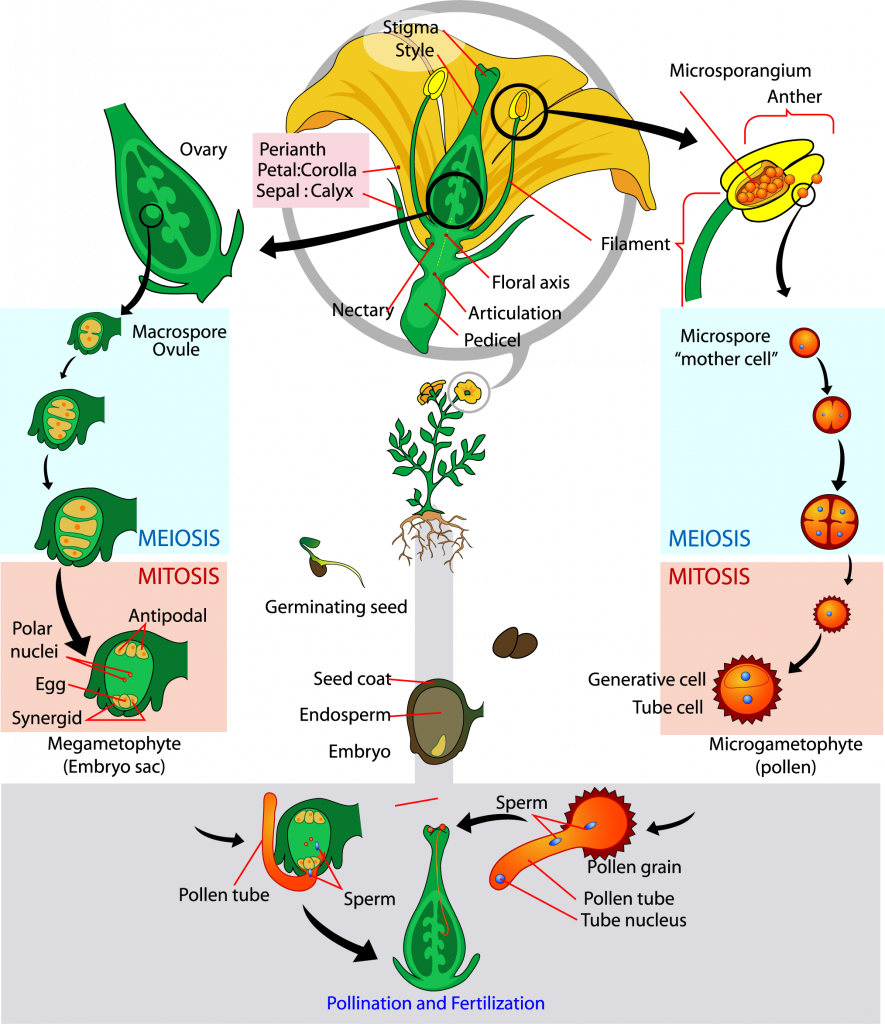
Plant growth is divided into two generations that are diploid (2n) and haploid (1n). Higher plants (angiosperms) have a long-lived sporophytic generation that is the diploid sporophyte. The sporophyte is the growth you would easily recognize as a plant. Through the process of meiosis, the sporophyte produces haploid spores in the flower. The spores are the gametophytic generation. Meiosis occurs in the male flower parts to produce pollen (represented by the green circle) and the female floral organs produce egg cells (represented by the white circle). Spores grow by mitosis producing more haploid cells, this is the gametophytic generation. We get a brief glimpse of the gametophytic generation when pollen is released from the flower, the female gametes are hidden from direct view in the ovary. When the haploid gametes (male pollen and female egg cells) unite they reform the sporophytic generation producing a diploid (2n) zygote. The zygote grows into the embryo of the seed and eventually into the plant we see. Lower plants, mosses and ferns that are not flowering plants, also alternate generations, but the gametophytic generation is longer lived and separate from the sporophytic generation.
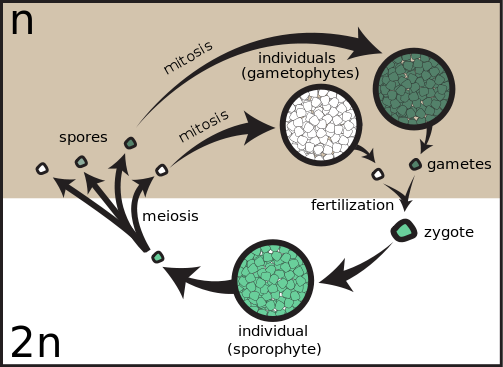
The plant doesn’t magically transition to being haploid, but instead particular parts of the flower in the androecium and gynoecium develop and protect a limited number of haploid cells, called the male gametophyte and female gametophyte. A later chapter addresses how the male and female gametophyte include the haploid egg and sperm cells that must unite to form the diploid embryo in seeds. For now, know that meiosis is the gateway into the haploid phase. Meiosis is the type of cell division that starts with diploid cells and results in haploid cells. Without meiosis there is no egg and sperm, and thus no sexual reproduction.
Propagation and natural selection
From the natural selection perspective, how do asexual and sexual reproduction differ?
In asexual reproduction, the plants are genetic copies of the parent plant. Cell division is strictly mitosis. Except for rare mutations, the resulting progeny are identical to the parents. The fitness of the progeny will mirror the fitness of the parent. The downside of this type of propagation is that there is no genetic variance among progeny that might result in selection for plants that have greater fitness than the parent for characteristics such as increased cold hardiness, drought tolerance, or disease resistance. The upside is that if the parent has high fitness to begin with (and it must have had reasonable fitness to reach reproductive age), all progeny will also have that high level of fitness. If the environment remains the same as it was for the parent, the progeny will stand a good chance of reproductive success. But if the environment changes, the fitness of the progeny may no longer be optimum.
In sexual reproduction, since one gamete comes from the male parent and one from the female, and because in a population of cross-pollinating wild plants there are many potential parents, each with different genotypes, there are many potential genetic combinations of male and female gametes. Not only are the plants producing the gametes each genetically different, but each gamete from each plant is potentially unique. The many combinations of male and female gametes, and the uniqueness of gametes from the same plant, result in a substantial genetic variation among the progeny of plants that sexually reproduce. Some of these progeny will have greater fitness than others, and will be favored by natural selection — some will survive to reproductive age and have more progeny than other plants, while the rest will either not survive to reproduce or, if they do reproduce, it will be with low frequency. The DNA of the fittest plants will thus be represented more frequently in the next generation of plants than the DNA of the least fit plants, which may never survive to reproduce and pass on their DNA. This is the process of natural selection. The DNA of reproductively successful parents is passed on the next generation, while the DNA of reproductively unsuccessful parents is not.
Sex generates genetic variation. Genetic variation, generated by meiosis and sexual reproduction, is the fuel for the engine of natural selection.
Ploidy review
To review: if you count the number of chromosomes in a somatic cell, for instance a root tip cell, you will find that there is always an even number. These are diploid 2n cells that arose from mitotic cell divisions tracking all the way back to the zygote that formed the embryo of the seed. Listed below are the numbers of chromosomes found in somatic, diploid, 2n cells of a few commonly grown plants. Note that the number of chromosomes is even, never odd, and that it doesn’t imply anything about the size or type of plant:
- Corn = 20
- Rice = 24
- Soybean = 40
- Green bean = 22
- Tomato = 24
- Potato = 48
- Apple = 34
- Rose = 14
Diploid cells always contain an even number of chromosomes because there are two copies of each chromosome, one contributed by the male sperm and one by the female egg. If you number each type of corn chromosome 1 through 10, there would be two 1s (a maternal and a paternal), two 2s, etc. Recall that the two (donated from the male and female) versions of the same chromosome in a diploid cell are called homologous chromosomes or homologs. In a diploid cell like corn where 2n=20, there are 10 pairs of homologous chromosomes.
Also recall that the number of chromosomes in a gamete is half the number of chromosomes found in a somatic cell of the same plant. The gamete cells are haploid, abbreviated n. There may be an even or odd number of haploid chromosomes, depending on the diploid chromosome number. Beans have a diploid number of 22, so the gametes have an odd number of chromosomes (11). Tomato has a diploid number of 24, so the gametes have an even number of chromosomes (12).
When two gametes fuse and form a zygote, the zygote has the 2n chromosome number restored. From then on, the cell divisions that allow a plant to grow from zygote to full size are all mitosis, and all the cells are copies of the zygote formed by fusion of the two gametes.
Review questions
- In what sense is meiosis the gateway into the haploid or gametophytic stage of alternation of generations?
- Why does a diploid plant cell always have an even number of chromosomes?
- A diploid rose cell has 14 chromosomes. How many pairs of homologous chromosomes will you find in that diploid cell?
Meiosis mechanics
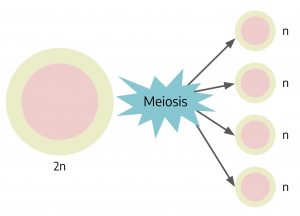
Meiosis starts with a diploid cell and results in haploid (n) cells that we could correctly call spores. In the illustration above, note that starting with one diploid cell and meiosis yields four haploid cells.
Below are the stages of meiosis. It won’t be difficult to memorize them because you already know the stages of mitosis, and meiosis builds on mitosis. The illustration shows a hypothetical species with two pairs of chromosomes (2n=4) in the starting cell and n=2 in the resulting gamete cells. Meiosis has two chromosome divisions, so the stages are labeled I for those stages associated with the first division (e.g., Metaphase I) and II for those associated with the second division (e.g., Metaphase II).
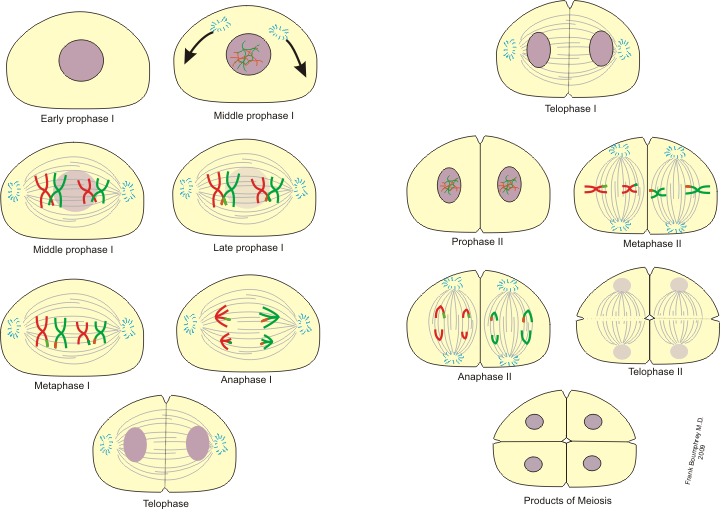
As in mitosis, the cell division process starts when the chromosomes replicate in the S phase of interphase.
Prophase I — the nuclear membrane disintegrates, and we see that the chromosomes have already replicated (in “S” of interphase) so that the now condensed chromosomes are made up of two sister chromatids attached at the centromere. New, however, is that in this stage the homologous chromosomes pair up and form structures called tetrads because they are groupings of four sister chromatids (two sister chromatids per homolog). This process of pairing and tetrad formation promotes chiasma (seen under the microscope as the point where sister chromatids of homologs lay over each other, forming an “X” shape) and crossing over between sister chromatids of homologous chromosomes. Crossing over results in an exchange of DNA between homologs and is another contributor to genetic variation in the gametes and resulting organisms.
Reread the preceding paragraph, making sure you understand how homologs pair, form chiasma, and cross over between sister chromatids. This type of pairing of homologs and subsequent chiasma formation doesn’t happen in mitosis — a very important and essential difference between the two types of cell division.
Metaphase I — the tetrads are lining up on the metaphase plate, ready to divide. Recall that they are called tetrads because they are made up of four sister chromatids.
Anaphase I — the tetrads divide and homologs go to opposite poles. Note that sister chromatids stay intact and the centromeres do not divide yet. It is the homologous chromosomes that separate at Anaphase I. The sister chromatids may differ in some places along their arms due to crossing over that causes exchange of DNA between homologs.
Telophase I — the nuclear membrane reappears to separate the products of the first division.
The DNA relaxes in Interphase I, but no replication occurs. DNA condenses again in Prophase II, and we can see the chromosomes.
Metaphase II — the chromosomes (which are in the form of sister chromatids still connected at the centromere) line up at the metaphase plate, as in metaphase of mitosis.
Anaphase II — also like anaphase in mitosis, the centromeres split and sister chromatids are pulled to opposite poles.
Telophase II — nuclear membrane reforms, cytokinesis takes place, just like the telophase of mitosis. Note that the “II” stages of meiosis are just like the corresponding stages of mitosis, making them easy to remember.
Review questions
- Do the chromosomes replicate prior to meiosis?
- What happens during crossing over?
- The illustration above shows that some of the sister chromatids are combinations of red and blue rather than being all red or all blue. What does that represent?
- What separates in Anaphase I?
- What separates in Anaphase II?
Division
Watch this video for a detailed explanation of division (8:19)
Memorizing this process helps you focus on clearly understanding the mechanics of the process, and recognize how it is that meiosis results in four n haploid cells instead of the two 2n cells that result from mitosis. You get four n haploid cells because the initial cell undergoes two divisions. The cell first divides into two nuclei, then those two divide again into four. The chromosome number drops from 2n in the original cell to n in each of the four haploid cells because the number of sets of chromosomes is reduced from 2 to 1 (that is, homologs separated to opposite poles) in the first meiotic division, and then the sister chromatids separated in the mitosis-like second meiotic division. There was chromosome replication before the first division, but no replication before the second division.
Here’s a summary of what is dividing and when:
Two divisions:
Homologs separate in Anaphase I. Centromeres holding the chromatids do not split.
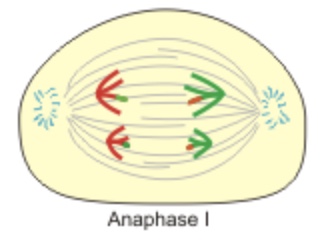
Chromatids separate in Anaphase II. Centromeres holding the chromatids do split.
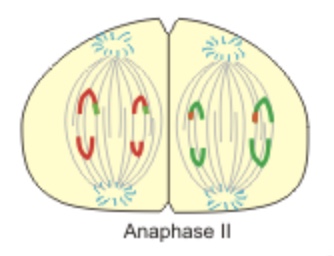
Genetic variation among gametes
Watch this video to see how meiosis contributes to genetic variation. (9:26)
Each gamete ends up with one of the homologs of the pair, not both.
- Imagine that there are two or three or even 30 pairs of homologous chromosomes. Each homolog pair making up the tetrad in Metaphase I separates in Anaphase I. One homolog from the pair heads to one pole and the other heads to the opposite pole. Each pair of homologs moves independently of all the other homolog pairs that are also separating. That is, all the paternal-source chromosomes making up the homologous pairs don’t go to one pole and all the maternal-source go to the other pole (this is possible, but since it would be by chance the probability is very low). Instead, paternal-source homologs of some chromosomes and maternal-source homologs of the other chromosomes are pulled to the poles, so that eventually there is a mix of maternal- and paternal-source chromosomes in the gametes, and that mix of maternal and paternal is generally thought to be random.
- This principle, where homologs move to poles independently, is called independent assortment, and leads to differences in the genotype of the gametes — one source of genetic variation among gametes.
Crossing over and exchange of DNA between homologous sister chromatids in Prophase I is another source of variation in gametes. If two cells in the sporangia are undergoing meiosis, the crossing over in each cell will probably happen in different places on the chromosome in each cell, resulting in exchanges taking place at different locations on the DNA backbone so that the gametes resulting from different cells going through meiosis will all be unique.
What is crossing over?
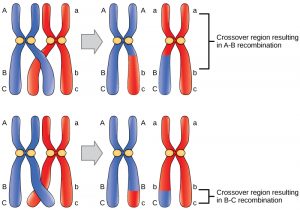
The illustration above shows a tetrad where the red and blue homologous chromosomes of the same chromosome type are pairing. Each homolog here consists of two sister chromatids joined at the centromere. Note that the chromosomes have already replicated in interphase prior to the start of Prophase I.
In Prophase I, homologous chromosome pairs come together (synapsis).
Arms of sister chromatids from different homologs overlap (chiasma) and exchange DNA (crossing over).
Note that all four of the resulting sister chromatids are now genetically different, with each potentially having a different DNA sequence.
Summary
- Meiosis is a type of cell division that starts with a diploid, 2n cell.
- The process includes two chromosome divisions and produces four haploid, n cells.
- The haploid cells are genetically different from each other due to crossing over in Prophase I and independent assortment in Anaphase I.
- Homologs separate in Anaphase I while sister chromatids separate (the centromeres divide) in Anaphase II.
Review questions
- Why does independent assortment during meiosis contribute to genetic variability of gametes?
- Why does crossing over contribute to genetic variability of gametes?
Haploid single cell produced by meiosis in the sporangium of a diploid sporophyte.
Groupings of four sister chromatids.
Point where sister chromatids of homologs lay over each other forming an "X" shape.
Exchange of arms of DNA between sister chromatids of homologous chromosomes that can take place at the point of chiasma formation.
Structures in the androecium and gynoecium where meiosis takes place and the gametophyte generation develops.