1.2 Science and Experimentation
Learning objectives
By the end of this section you will be able to:
- Describe why science is considered a discipline of philosophy.
- Summarize the four basic types of experiments.
- Apply the principles of experimental design in this course and in your daily life.
Thinking about science
The primary goal of this section is to help you think about the nature of science. You might be taking this course to fulfill an undergraduate requirement for a biology course with a lab. This course fulfills that requirement because we investigate the process behind using science as a way of learning about the natural world around us. If you’re starting down the path to becoming a plant scientist, understanding the nature of science will be essential for you in your career
Regardless of whether you’re going to pursue a career as a scientist, now is a good time reflect on the nature of science, and to understand how scientific thinking can become a strategy for resolving many issues that you confront during daily life.
Watch this video about connecting science and experimentation to real life:
Scientific inquiry
While “science” is a word commonly used in our culture, in popular use it is rarely spoken of as a philosophy. By identifying science as a philosophy we are taking an epistemic view, one focusing on how knowledge is acquired.
At its core, science is a mode of inquiry: a way of acquiring new knowledge about the world around us and a strategy for understanding the inner workings of elements in that world. Scientists believe that if we follow the principles of this philosophy we will continue to expand our knowledge about how things work in the world around us. This systematic approach is called the “scientific method.”
There are two key steps in the scientific method:
- Hypothesis building through reflective observation.
- Hypothesis testing through experimentation.
A “hypothesis” is a question or proposed explanation made on the basis of limited evidence and used as a starting point for experimentation. Experimentation is commonly equated with science—rightly so, because hypotheses evaluated on the basis of evidence generated through experiments. Experimentation, however, isn’t the whole story. Science—including the development and testing of new hypotheses—is also a creative endeavor.
Watch this video about scientific inquiry:
Scientific inquiry has generated a vast body of knowledge about the world around us. Your school science classes might have required you to memorize facts and relationships, and pay attention to detail. Sometimes such memorization leads students to believe that science is just an accumulation of facts rather than the process behind discovering all of that information.
Scientific discovery builds on what is already known. Even the most accomplished scientists initially approach a problem by learning what is already known. Armed with that information, they then apply their own creativity to form new hypotheses about something they have observed, and design experiments to test those hypotheses. They also communicate their results publicly so that others can benefit from their work and have the opportunity to challenge conclusions. In this way, science builds on itself.
The foundational knowledge you learn in science classes prepares you to develop and test hypotheses and to make new discoveries of your own. While a good memory may help you pass a science classes, you will absorb a body of knowledge more effectively when you learn how facts fit and work together in systems rather than learning through the brute force of memorization.
In this section we work from the point of view that science is a way of acquiring knowledge—a mode of inquiry—and that this mode of inquiry follows a process called the scientific method. Those who follow the philosophy of science:
- Use it to understand how the natural world works.
- Start by learning what is already known.
- Carefully observe the subjects of their scientific inquiry and look for details about form, function, and interaction with the environment.
- Develop hypotheses about the inner workings of natural phenomena not yet understood.
- Test their hypotheses by making observations, conducting experiments and collecting and evaluating evidence.
- Communicate with others about their hypotheses, experiments, and the outcomes of their studies so that others can repeat, validate, and build upon their work.
Although science is typically used to understand how the natural world works, it is also regularly applied to the development of new technologies that are based on these natural phenomena and to the solving of problems associated with the natural world.
Putting the scientific method to work
As noted, the scientific method relies on building hypotheses and then testing them through experimentation. In the lab section of this course you will develop hypotheses about the effects of various treatments on propagation success and then conduct experiments to test those hypotheses. Because experimentation is such a key component of the scientific method, we’ll spend time characterizing and examining four types of experimentation and explore whether they are part of the scientific method. While each is valuable when applied in the right circumstances, only one clearly follows each step of the scientific method to uncover new knowledge about the natural world.
Types of experiments
The types of experimentation we will cover are:
- Demonstration
- Evaluation
- Exploration
- Discovery
Demonstration experiments
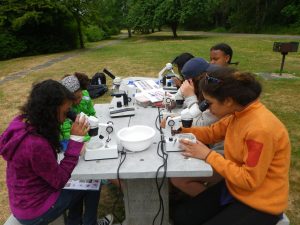
Demonstration experiments are a classic method used in educational settings to help students learn and understand known relationships already discovered by others. Learners will usually have had prior exposure to the relationships through preliminary observations, lectures, reading, and discussions, and will have some sense of what the experimental outcome might be.
Good demonstration experiments actively involve the learner, who manipulates the experimental materials, applies the treatments, and observes the outcomes, then gathers, analyzes, and interprets the resulting data. Poor demonstration experiments, in contrast, make learners only passive witnesses to something done by an expert at the front of the classroom.
In the plant propagation labs for this course, you will be actively engaged in demonstration experiments. Although you won’t be creating new knowledge, the knowledge will likely be new to you. The hands-on experience of conducting the experiments will help you to learn the concepts more effectively than if you only read a textbook or listened to a lecture. The techniques you learn and use in demonstration experiments often contribute to the learning experience as much as the relationships revealed at the experiment’s conclusion. Employing these techniques will help you gain an understanding of many biological functions, such as the production of adventitious roots and mechanisms for seed dispersal.
While demonstration experiments are valuable for actively learning a body of scientific knowledge previously discovered and communicated by others, the experience is specifically orchestrated for teaching and learning, not for the discovery of new information. Yet since the knowledge is new to the learner, it can still bring the joy of personal discovery and a sense of accomplishment.
In summary, demonstration experiments:
- Are designed for teaching and learning.
- Address relationships that may be new to you, but are otherwise known.
- In their best forms, actively involve the learner.
- May emphasize experimental techniques, in addition to outcomes, as part of the learning experience.
- Are not the types of experiments that are at the core of practicing science as a way to uncover new knowledge.
Evaluation experiments
Evaluation experiments are designed to help us make decisions, and to choose from a number of options. They might, for instance, help us determine the efficacy of a new treatment relative to a known treatment, or decide on further experimentation. An evaluation experiment will highlight a compound, a technique, a piece of equipment, or an organism, and will include a control and/or other alternatives.
Evaluation experiments are common in horticultural and agronomic research, where the purpose of the experiment is to identify, for example, the best cultivar, production method, pest control, fertility regime, or light intensity for growing a crop. Correct experimental design is crucial for assuring that conclusions from the experiment are meaningful and credible.
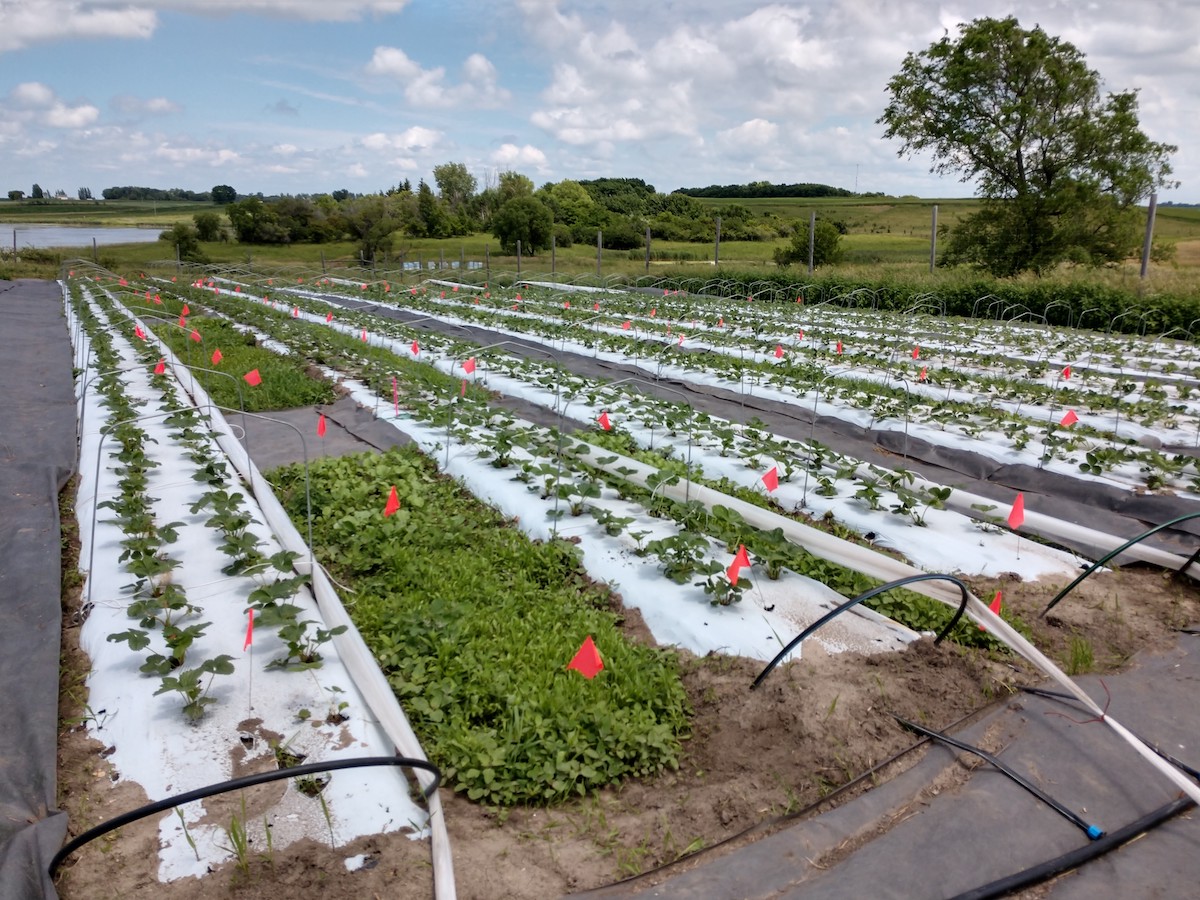
These experiments are typically used in the development of new technologies to identify the best method for the desired purpose (e.g., which pesticides are effective against the target insect, but not harmful to non-target insects). They are not used to discover new knowledge about how the world works, as they typically don’t advance our understanding of the natural world. The information from an evaluation experiment might, however, point the way to additional experimentation that does help us discover new knowledge. This is particularly true if the outcome of an evaluation experiment is unexpected or novel.
In summary, evaluation experiments:
- Are used to help in decision-making.
- Help users choose a winner or determine efficacy relative to other alternatives.
- Are commonly used when evaluating and recommending horticultural production methods.
- Can be useful in solving problems and developing technologies.
- Require proper experimental design (e.g., comparison to a control) for credibility and meaningfulness.
Exploration experiments
Some scientists specialize in observing and cataloging nature, and some aggressively search for previously unknown phenomena. In the botanical realm, such scientists study the diversity of organisms within habitats, discover new species, or are in other ways very skilled in “seeing” nature. Explorer-scientists recognize and appreciate detail and can identify the enormous diversity among plants by comparing characteristics that might be overlooked by others. They may also have the capacity to recognize possible interrelationships among organisms and with habitats, making their work particularly important to science. They might notice, for instance, that a particular species of plant is commonly found in wet areas but not in dry, or that a particular vegetable tastes sweeter when grown at higher altitudes than when grown closer to sea level. They don’t confirm the cause of these relationships, but are the first to notice them.
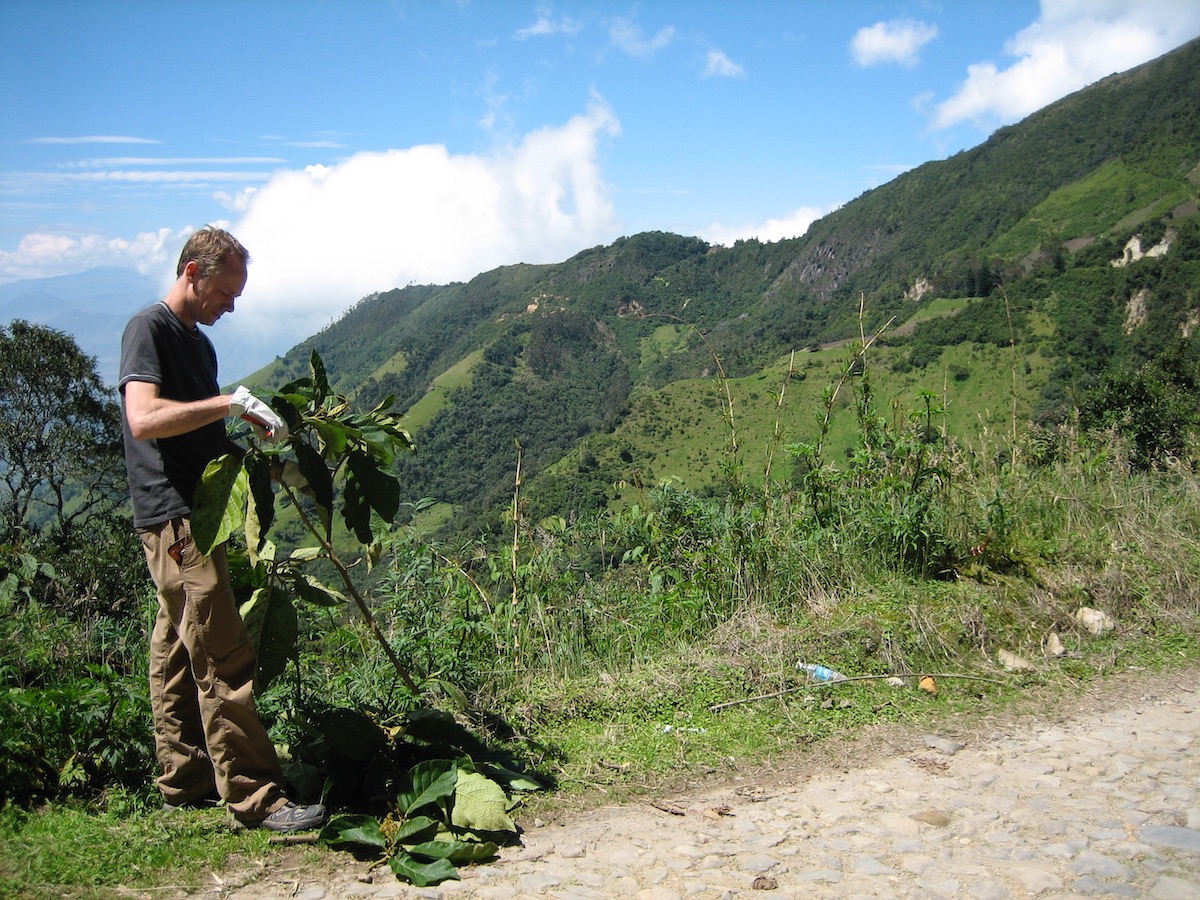
Explorers’ observations are essential to stimulating the development of sound, testable hypotheses. The possible relationships they propose must be tested to determine whether those relationships actually exist, or are artifacts of other effects. Explorers help develop hypotheses, but the work of exploration, cataloging, and seeing possible relationships don’t prove or disprove the hypotheses or necessarily generate new knowledge about relationships. The work does, however, result in new information about the existence of the object or phenomenon itself. An exception is exploration done to test a hypothesis, such as a mission to test the hypothesis that a particular type of ecosystem is required for reproduction of a particular plant species.
Scientists must resist jumping to conclusions based on exploration and observation alone. If you see two people together many times, for example, you might conclude that they are a romantic couple, when in fact they are brother and sister. Relationships hypothesized as a result of exploration and observation must be experimentally tested before they are accepted or rejected.
Exploration experiments uncover new things, many of which can be exciting and eventually change our view of the world. While one of their greatest values is that they lead to the development of new and stronger hypotheses about how the world works, they go so far as to test those hypotheses or fully engage in the cycle of knowledge generation associated with the scientific method. Additional experiments based on this new information are required to put this new information in context and to advance our understanding of how the natural world works.
In summary then, exploration experiments:
- Focus on detailed observation of organisms and habitats.
- Increase our knowledge of the natural world.
- Identify potential relationships that need to be tested.
- Are essential to sound and testable hypothesis-building.
Discovery experiments
Discovery experiments are central to the use of the scientific method in tasks ranging from problem solving to the discovery of new knowledge. They focus on uncovering new relationships and solving problems, follow the scientific method, test hypotheses and their predicted outcomes, and utilize a careful design in order to maintain meaningfulness and credibility.
The similarity between the scientific method and Kolb’s Experiential Learning Cycle is not an accident. The scientific method is a practical strategy based on how we sense and experience the world around us and used to solve problems encountered during those experiences.
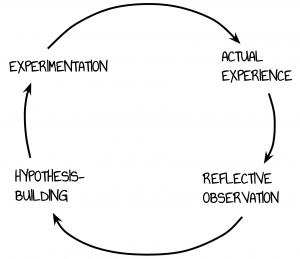
The diagram above illustrates a combination of the scientific method and Kolb’s four-step experiential learning, describing a cyclic process for solving problems that can be applied to disciplines as diverse as molecular biology, global warming, and even appliance repair. While you might initially think that appliance repair doesn’t belong in that list, the difference is one of application, not method. Though far removed from the esoteric scientific discoveries we associate with scientific method, appliance repair follows the same steps. Appliances are often, and quite literally, boxes, where you don’t know what is going on inside. But what’s going on inside is knowable, and through that knowledge comes repair.
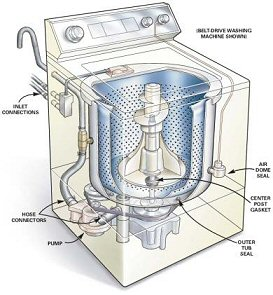
The learning/problem solving/scientific process could theoretically start anywhere in Kolb’s cycle. But it will likely start with a problem that needs to be solved, something you don’t understand but would like to know more about. You become aware that there is a problem or that you lack understanding because you have an experience where you observe something and then step back and said, “I wonder how that works,” or perhaps, “why is that broken?” Through observation you develop a sufficiently adequate description of the problem to start doing some research on what is already known.
With a good description of the problem in hand, you can begin to review what is known through the work of others, and think about what might be going on in your situation and how your new understanding can be applied to the problem. This is “reflective observation.” It isn’t just sitting back and thinking in a vacuum. You need raw material for your mind to work on, and that only comes through the tough task of gathering and engaging with the background information. There is a very important quiet phase in this process when you let your mind assemble and sort through ideas until alternatives begin to emerge that might lead to a solution. Talking with others and sharing ideas is an important part of this quiet phase.
Sometimes the alternatives are no- brainers (blown fuse?), and sometimes they’re more creative (residue from the wrong detergent gunking up the water level sensor?). Regardless of their simplicity or complexity, these become hypotheses that need to be tested. The hypothesis-building stage includes both a statement of how something works or why it isn’t working, and predictions about what might happen if the hypothesis is true. In appliance repair, for example, the prediction will likely be that the appliance will function normally. In horticultural molecular biology, it might be that you will see accumulation of a particular type of fatty acid in the cotyledons.
You put the hypothesis to the test by designing an experiment that assesses whether your predictions were right. If the outcome doesn’t match your prediction, you reject the hypothesis (the fuse was ok, so that wasn’t the problem). If the outcome does match your prediction, you tentatively accept the hypothesis pending further observation (when the fuse was replaced the washing machine worked again, so it might have been a blown fuse, but on the other hand maybe it was just because the motor had time to cool down). As with evaluation experimentation, experimental design is important in assuring that the conclusions from the experiment are meaningful and credible.
Experimentation leads to new experiences and an incremental increase in knowledge, and then the cycle begins again.
In summary, discovery experiments:
- Focus on uncovering new relationships and solving problems.
- Follow scientific method.
- Test hypotheses and their predicted outcomes.
- Utilize a careful design in order to maintain meaningfulness and credibility.
Summary
Of the four types of experiments, only the discovery experiments are core to the process of science in the narrow sense of being a way of acquiring new knowledge. The other three types of experimentation are still important; demonstration and evaluation experiments are valuable for learning and decision-making and for technology development, and exploration experiments are essential for developing testable hypotheses. But discovery experiments are core to science.
Remember: the methodology of effective washing machine repair, when applied to what is unknown about the physical world, is the methodology of science. It’s not esoteric; it’s good appliance repair.
You might argue that, when applied to a broken washing machine, a discovery experiment results in knowledge that is probably already known by those skilled in appliance repair, so it isn’t really new knowledge about how the world works. That’s a fair criticism. Use of the scientific method can result in new knowledge about how the world works, but whether it uncovers new knowledge depends on the object of experimentation.
Review questions
Experimental design
The methods for designing experiments are carefully studied and often discipline-specific. Methods used in molecular biology, for instance, will be somewhat different from those used in chemistry or in field evaluations of horticultural plants. There are, however, some generalizations we can make about good experimental designs.
Emphasize comparisons
Experiments include more than just one treatment. “Treatment” refers to the factor that you are varying in your experiment—for example, different cultivars of tomato, different fertilizers, or different amounts of light. Experimental designs incorporate comparison of treatments. You usually compare the treatments to one another and often to a control, which is either the application of no treatment or the application of a customary or standard level of treatment.
If you grow a particular type of tomato in your garden, and find that it produces tasty fruit, would you declare it to be the best tomato variety you could grow? Certainly not. You couldn’t even say with certainty that it was the best tomato variety you have ever grown (unless it is the only one you have grown). Next year, however, you could grow that tomato as your control, and grow two other varieties that your neighbors like, and compare fruit quality (appearance, flavor, yield, sugar content). You could then say something definitive about the three tomato varieties because you have compared them to each other after growing them next to each other in the same year and environment.
Replicate treatments
The same treatment is applied to more than one “experimental unit”—the object that receives the treatment. In the example above, the tomato plant is the experimental unit, and you would perhaps plant two or three seedlings of each tomato variety rather than just one. Think of a treatment as something like a fertilizer spread on a patch of land. The patch of land is the experimental unit, while the fertilizer is the treatment.
By applying the treatment to more than one experimental unit you can estimate the variation you get when two experimental units are treated the same, and compare this to the variation when experimental units are given different treatments. If the treatments actually differ in their effectiveness, you would expect the variation between experimental units given different treatments to be much greater than the variation between those given the same treatment. This is one of the fundamental ways in which experiments are statistically analyzed and treatments declared significantly different or not.
Randomize treatments
Once you know how many treatments you are going to apply, and how many replications you want, the product of these two quantities (# treatments × # replications) equals the number of experimental units you need. For instance, if you have three fertilizers you want to test, plus a control, you have four treatments. If you want three replications of each treatment, then you 4 treatments x 3 replications = 12 experimental units or patches of land where you will apply the fertilizers. The treatments will be randomly assigned to each experimental unit (patch of land). This is done using a random number table and is not just haphazard picking. Randomization helps minimize any bias you haven’t recognized in advance and controlled for in other ways.
Review questions
- What are two types of control treatments?
- Does increasing the number of replications increase the number of treatments or the number of experimental units?
- Can you think of an example of how randomization can protect against bias?
Process of scientific inquiry; it builds on what is known by testing hypotheses.
A very valuable method for actively learning the body of scientific knowledge that has been previously discovered and communicated by others; and it is specifically orchestrated for teaching and learning, not for the discovery of new information about the world around us.
Typically used during the development of new technologies to identify the best products for the desired purpose (eg. which pesticides are effective against the target insect, but not harmful to non-target insects), but are not used to discover new knowledge about how the world works so they typically don't advance our understanding of the natural world. Used to pick a winner from among a number of options.
Used to verify or regulate a scientific experiment by conducting a parallel experiment or by comparing with another standard
A plant variety that has been produced in cultivation by selective breeding. The term comes from combining the words 'cultivated' and 'variety'.
Process of planning an experiment to test a hypothesis.
An embryonic leaf in seed-bearing plants, one or more of which are the first leaves to appear from a germinating seed.
When the same treatment is applied to more than one experimental unit.
Act of randomly assigning treatments to experimental units using a random number table or computer-generated randomization to help minimize any bias that has not been recognized in advance and controlled for in other ways.