2.13 Adaptation, Fitness, and Diversity
What is fitness?
Of the four mechanisms of evolution (mutation, natural selection, migration, and drift), which mechanism consistently causes organisms to have more offspring? All four mechanisms can cause evolution, a change in the frequency of traits within a population. But which mechanism consistently causes the frequency of a beneficial trait to increase?
The answer is natural selection. Natural selection is the only mechanism that consistently increases fitness. What do we mean by fitness?
Fitness is the “currency” of evolutionary success. You may have heard the phrase “survival of the fittest.” A more accurate (though admittedly less catchy) statement would be “reproductive success of the fittest” because it is the number of offspring that determines an organism’s fitness, not the length of its life. A bird that lives longer than other birds, has more colorful feathers, and can fly further may seem like a winner. However, if the bird fails to reproduce, it will have lower fitness than other birds that do have offspring. Evolutionary “winners” are those individuals who are best represented, genetically, in the next generation.
Organisms with high fitness produce more offspring relative to others because they are better adapted to the environment. The traits that cause higher fitness are called adaptations.
Adaptations come in a variety of forms
Adaptations can be anatomical features, such as the strong tail fin of fish that cannot survive without strong swimming. The large ears of the desert fox is an adaptation that helps the fox dissipate heat in its hot environment. The modified leaves of the cactus, its sharp spines, are also adaptations for desert life; these spines limit the plant’s loss of water in its arid climate and serve as defense against potential plant predators. The plant-like features of the stick insect allow it to blend into its surroundings, an adaptation that confers protection against predation.
Biochemical features, such as proteins, or the genes that code for proteins, can be adaptations. Genes for egg-yolk protein, vitellogenin, are present and functioning (producing egg-yolk protein) in fish, reptiles and birds. These genes for egg-yolk protein are adaptations that distinguish the egg-laying birds.
Behaviors can be adaptations as well. A plant that orients its leaves to face the sun is responding in an adaptive way to its environment. A dog that barks at an intruder is exhibiting a protective adaptation, and the Cape ground squirrel that uses its own tail for shade is demonstrating a thermoregulatory adaptation. To see this tail in action as a parasol and an anti-predator device, check out the following video. The arctic tern, which travels up to 60,000 kilometers from its Arctic breeding grounds to the Antarctic, is implementing a behavioral adaptation that is energetically taxing but leads to reduced competition for resources, reduced predation pressure, and greater food availability.
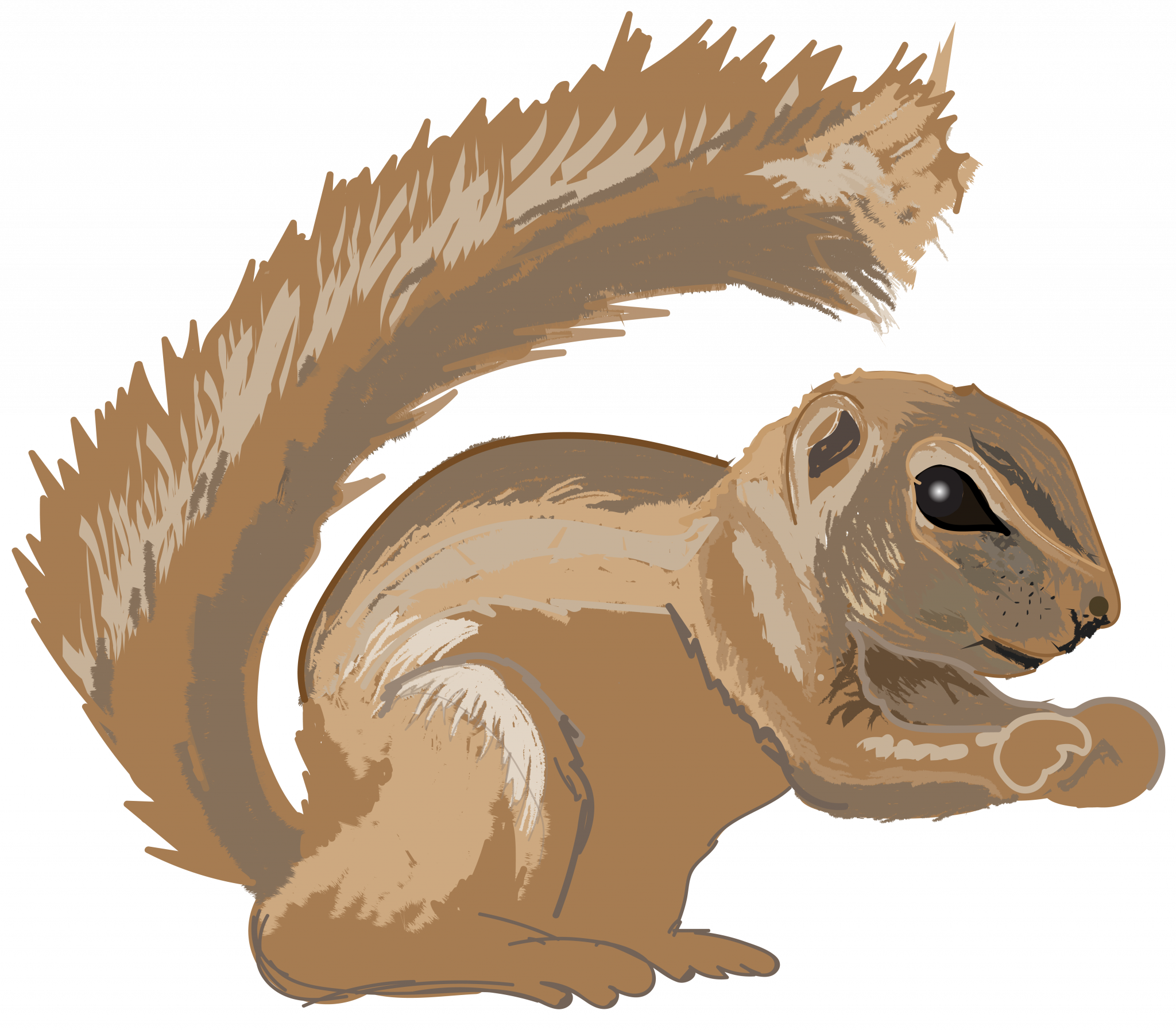
Adaptations are environment-specific
Adaptations are specific to the environment an organism lives in. If an individual with an adaptive trait were to move to a different environment, that trait may no longer cause high fitness, and it therefore would no longer be called an adaptation. For a trait to be considered an adaptation, it must have evolved because the trait is beneficial in the population’s specific environment. Take our earlier example of a species of frog that is green and lives in a lush, green environment. The green trait is an adaptation because it provides protection from predators. However, if there is an extreme drought, the leaves may turn brown. Now green coloration is no longer an adaptation.
The generation of genetic diversity is a product of evolutionary processes
Biodiversity is the variety of life. Evolutionary biologists quantify biodiversity at the population level by measuring the degree of genetic diversity within a population–the number and frequency of different gene variants. As we’ve discussed, mutation and migration typically increase genetic diversity, and natural selection and drift typically decrease genetic diversity (though there are some cases when natural selection increases diversity).
Ecologists often quantify biodiversity within an ecosystem as the number of species in a specific region. The process of evolution creates new species and causes diversity to increase. Over time, isolated populations of the same species can diverge so much that they are considered separate species. For example, an ancestral population of lizards, possibly from mainland South America, migrated to the Galápagos islands (in the equatorial Pacific Ocean) millions of years ago. After many generations of mutation, drift, and selection, the colonizing population was sufficiently different from the parent population to warrant classification as a different species. This pattern was then repeated throughout the islands, with mutation, selection, migration and drift all acting differently on different island populations. Thus, from one colonizing species, the archipelago now has several different species of what we call lava lizards. All of these lizards are endemic to the archipelago—that is, they are found nowhere else in the world (Figure 2.17).
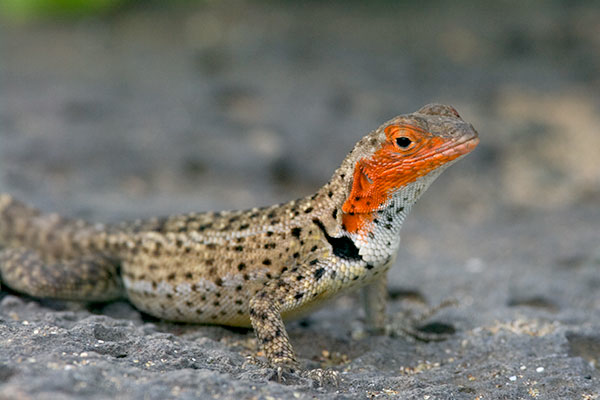
Why don’t humans have yolks?
The genes for producing vitellogenin, a gene involved in the production of nutrient-rich yolk, exist in non-functioning, or vestigial, forms in all mammals with the exception of the egg-laying mammals, the monotremes. (Monotremes have one functional egg-yolk gene and one non-functional egg-yolk gene.) These genes suggest a story about mammalian evolution. Namely, our mammalian ancestors began by nourishing our young with egg-yolk protein, like the modern-day reptiles and birds. As our ancestors developed the ability to lactate (i.e., feed our young with milk), mutations arose that rendered them unable to make egg-yolk protein. With the ability to feed offspring milk, the egg-yolk protein was no longer advantageous and therefore individuals with non-functional egg-yolk protein genes were not at a disadvantage anymore. Thus, a new group of organisms emerged without functional genes for egg-yolk production.
Content on this page was originally published in The Evolution and Biology of Sex by Sehoya Cotner & Deena Wassenberg and has been expanded and updated by Katherine Furniss & Sarah Hammarlund in compliance with the original CC-BY-NC 4.0 license.